Untersuchung und Entwicklung von verschiedenen elektrische Raumfahrtantrieben, Plasmaquellen, und Plasmawindkanälen.
- Atmosphärenatmendes elektrisches Antriebssystem (ABEP);
- Elektrostatischer Trägheitsschluss (IEC) als Triebwerk und für Plasmaanwendungen;
- Fremdfeldbeschleunigte MPD-Triebwerke (AF-MPD);
- Gepulste Plasmatriebwerke (PPT);
- Induktive Plasma Triebwerke (IPT, Helicon);
- Thermische Lichtbogentriebwerke (TLT);
- Wasserelektrolysetriebwerke;
- Entwicklung und Betrieb von Plasmawindkanälen zur Qualifikation von Werkstoffen für Hitzeschutzmaterialien und von numerischen Verfahren für Wiedereintrittsfahrzeuge, interplanetare Sonden und Raketenbrennkammern.
Kontakt
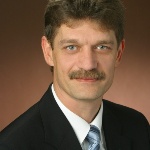
Stefanos Fasoulas
Prof. Dr.-Ing.Geschäftsführender Direktor, Professor für Raumtransporttechnologie, Dekan der Fakultät 6 - Luft- und Raumfahrttechnik und Geodäsie